Master Internship
When an intense laser pulse of femtosecond duration propagates through air, it gives rise to filamentation, a spectacular process where the beam contracts spatially to form a thin channel of light in which the intensity is maintained at ~1013 W / cm2. Filamentation is accompanied by the formation of a long column of short-lived plasma generated in the wake of the laser pulse. This column notably has the capacity to initiate and guide electric arcs of several meters with great reproducibility [1]. In recent years several applications based on plasma filaments have been proposed such as the laser lightning rod [2] and the radio frequency virtual antenna [3].
A crucial parameter for these applications (in particular for lightning triggering) is the lifetime of the plasma created by filamentation, which must be as long as possible. The aim of this internship will therefore be to characterize the parameters of the plasma produced by the femtosecond laser and to test different methods to increase this lifespan. To do this, different time-resolved spectroscopy, interferometry and imaging techniques [4] will be implemented. In particular, we will seek to develop a measurement of plasma temperature by Raman spectroscopy.
The candidate must have basic knowledge of optics or plasma physics, a good level of English and present solid academic references.
This internship will be paid and may lead to an extension into the thesis
Contact: aurelien.houard@ensta.fr
[1] B. Forestier, et al., “Triggering, guiding and deviation of long air spark discharges with femtosecond laser filament”, AIP Advances 2, 012151-13 (2012)
[2] A. Houard et al. Laser-guided lightning, Nature Photonics 17, 231 (2023)
[3] Y. Brelet, et al., Radiofrequency plasma antenna generated by femtosecond laser filaments in air”, Applied Physics Letters 101, 264106 (2012)
When an intense femtosecond laser pulse propagates in air, it gives rise to filamentation, a spectacular process in which the beam contracts spatially to form a thin channel of light in which the intensity is maintained at ~1013 W/cm2. Filamentation is accompanied by the formation of a long column of short-lived plasma generated in the wake of the laser pulse. This column is capable of guiding lightning [1] and producing secondary radiation from THz [2] to UV [3], which can be used for remote sensing applications in the atmosphere [4].
The recent development of laser technologies based on amplification in Yb:YAG crystals has made it possible to significantly increase the power of femtosecond lasers [5]. This type of very promising laser source was used by our team for the first demonstration of lightning guidance by laser filamentation [1], but the significant thermal effects that appear at this power are still poorly understood and very few studies of filaments have been carried out at this wavelength.
This internship will focus on the detailed characterization of the plasma produced in air by a 200 mJ, 800 fs laser pulse at 1.03 µm and 515 nm. We will also evaluate the effect of the laser repetition rate on the filamentation and characterize the different radiation produce by the filament at 1.03 µm.
Candidates should have a basic knowledge of optics or plasma physics, a good level of English and solid academic references.
This internship will be remunerated and may lead to an extension into a Ph.D thesis.
Contact: aurelien.houard@ensta.fr
Publications by the team related to the subject
[1] Laser-guided lightning, Nature Photonics 17, 231 (2023)
[2] White-Light Filaments for Atmospheric Analysis, Science 301, 61 (2003)
[3] Conical Forward THz Emission from Femtosecond-Laser-Beam Filamentation in Air, Phys. Rev. Lett. 98, 235002 (2007)
[4] Lasing of ambient air with microjoule pulse energy pumped by a multi terawatt IR femtosecond laser, Opt. Lett. 39,1725 (2014)
[5] Ultrafast thin-disk multipass amplifier with 720 mJ operating at kilohertz repetition rate for applications in atmospheric research, Optics Express 28, 30164 (2020)
Abstract : Laser-plasma acceleration makes it possible to reduce the size of electron accelerators
by 3 to 4 orders of magnitude, for societal and scientific applications. This acceleration concept is
based on focusing an intense laser pulse in a light gas that turns into a plasma. The laser pulse
expels the electrons out of its path and creates an ion cavity in its wake. Due to the complete
separation of charges, the fields in the cavity have amplitudes out of reach of other technologies.
Electrons injected in the cavity can thus gain very high energies on short distances.
Tremendous efforts have been made over the past three decades to master this approach and
produce electrons with energies up to about 10 GeV, but little attention has been paid to the
utility of relatively low-energy electrons. However, laser-plasma accelerators can efficiently
produce beams of low energy, high charge, high divergence, and micrometer source size that are
of great interest for industrial and medical applications. We recently optimized the accelerator for
these applications and observed that the beam divergence increases sharply with the charge.
While a broad divergence is beneficial for many applications, the use of the beam becomes too
complex beyond a certain level. We recently had an idea to reduce the divergence, while
retaining the charge: temporally or spatially separate the laser pulse into multiple pulses of lower
energy that each individually accelerates an electron beam. The electron beam thus produced has
the same charge as the beam generated with a single laser pulse but a lower divergence and a
longer total duration.
The objective of the internship is to carry out the first demonstration of this approach. This will
involve installing the device to create the pulse train, carrying out the experiment, and comparing
the results to the reference case. Numerical simulations could complete this work. The internship
could continue with a Ph.D. during which the student will continue to improve the source for
societal applications (testing new approaches). Depending on the student’s taste, the Ph.D. could
also address the theory.
Possibilité de thèse : Oui Pursuing into PhD : Yes
Contrat-financement probable / Expected contract-funding : CIFRE grant
Contact : Cedric Thaury
Abstract: As ionized media, plasmas are not subject to electrical breakdown and can thus withstand very high amplitude
electric fields. This property is at the basis of laser-plasma accelerators. An intense laser pulse is focused into a light gas
that turns into a plasma. The laser pulse then expels the plasma electrons out of its path and creates an ion cavity in its
wake. The electric fields in this cavity are 3 to 4 orders of magnitude stronger than those obtained in conventional
accelerators. Therefore, we can accelerate electrons to energies of a few giga-electronvolts (v>0.9999999c) in just a few
centimeters, whereas this would require hundreds of meters with conventional techniques.
The maximum energy of these so-called wakefield accelerators is ultimately limited by the dephasing between the electron
beam and the accelerating field. This dephasing comes from the velocity difference between the accelerating field and the
electron beam, which causes the latter to leave the accelerator field after an acceleration of typically a few centimeters. This
limitation could, in principle, be removed by controlling the velocity of the accelerating plasma wave, which requires the
generation of an intense laser pulse that propagates in a vacuum at a superluminal velocity (> c). We recently produced
such a pulse experimentally and numerically showed that it could dramatically increase the energy of the electrons produced
through laser-plasma acceleration. However, many questions remain open: how to inject electrons into this accelerator, how
to ensure that the laser keeps its unique properties in the plasma over long distances, and what is the energy efficiency of
this accelerator…?
The internship objective is to study the propagation of a superluminal and ultra-intense pulse in a plasma using a numerical
code developed in the laboratory. The results could be, at least partially, compared to analytical calculations. The internship
could continue with a PhD during which the student will study superluminal laser-plasma acceleration using Particle-In-Cell
simulations. Complex spatiotemporal couplings will be introduced to optimize the propagation of the laser or to locally slow
down the pulse and thus control the injection of electrons into the accelerator. The student will also study the energy
efficiency of this new accelerator concept using analytical and numerical approaches. Finally, he/she may explore the
potential of ultra-intense superluminal pulses for other applications.
Contrat-financement probable / Expected contract-funding : IPP grant
Contact : Cedric Thaury
Thematic:
Quantum electrodynamics, or QED, is the physical theory that unifies electromagnetism and quantum physics to describe how light and matter interact. It is considered how one of the best demonstrated theories in its linear regime through the measurement of the fine structure constant with unprecedented accuracy. However, it is in the so-called ‘strong fields’ regime, when the light intensity becomes extreme, that QED reveals its most amazing predictions such as the generation of matter from vacuum in the form of electron/positron pairs. These extreme regimes have been studied theoretically for more than a century but have never been reached experimentally.
The recent development of a new generation of lasers which deliver femtosecond pulses with a power of several PetaWatt, makes it possible to develop experimental strategies for achieving the QED strong field regime. The most commonly considered strategy to reach it is to achieve the collision of a relativistic electron beam with an ultra-intense laser field [1].
The strategy that will be studied during this PhD is the so-called ‘Doppler boost’ [2]. The ultra-intense laser pulse focused at a few 1022W/cm2 on a solid is converted to extreme ultraviolet (XUV) by relativistic Doppler effect during reflection onto a plasma mirror. The XUV beam, of much shorter wavelength, can be focused on a focal spot much smaller than the laser which has the effect of multiplying by 3 orders of magnitude the light intensity. The interaction of any type of matter (gas, solid, relativistic electrons) with this XUV pulse is then in the highly non-linear QED regime of strong fields [3].
Objectives :
The objective of the thesis is to generate ‘Doppler boosted’ pulses to obtain the highest light intensity ever reached on Earth, of the order of 1025W/cm2 and to use them to carry out the very first QED experiments in the regime of strong fields. Two types of experiments will be carried out: – The collision of the boosted Doppler beam with relativistic electrons. This experiment will be carried out at the Applied Optics Laboratory. – The interaction of the boosted Doppler beam with a solid. These experiments will be carried out on the largest laser infrastructures in the world: APOLLON, BELLA, ELI. A first experiment on the BELLA laser installation located in Berkeley, California, is being prepared for December 2024.
The objective of the internship is the theoretical and experimental understanding of the generation of Doppler boosted pulses and strong field QED, as well as the preparation of the different experiments with the design of several experimental diagnostics such as a XUV spectrometer, a Gamma beam spectrometer, and an electron/positron spectrometer, and the preparation of the experimental chamber located at the Laboratory of Applied Optics.
An experience on the Petawatt APOLLON laser installation will also be part of the internship in May 2024 to gain experience on this type of large laser infrastructure.
Profile of the candidate:
The aim of the proposed thesis is to perform very high impact experiments in the field of physics with the first experimental observation of QED phenomenon in strong field.
To achieve such objectives, the candidate must be passionate about research and have a high capacity and autonomy of work. Pragmatism is also an essential quality for an experimental thesis. You must obviously be prepared to travel abroad for several weeks. Finally, sense of humor for long evenings in the experimental rooms is always welcomed by all colleagues.
Diploma: Master 2 research in physics
Important to know: Python, Matlab
Pursuing into PhD : Yes
Expected contract-funding ? Yes
Contact : Adrien Leblanc
Biobliography
[1] Lobet, M, et al. “Generation of high-energy electron-positron pairs in the collision of a laser-accelerated electron beam with a multipetawatt laser.” Physical Review Accelerators and Beams 20.4 (2017): 043401.
[2] Vincenti, H. “Achieving extreme light intensities using optically curved relativistic plasma mirrors.” Physical review letters 123.10 (2019): 105001.
[3] Fedeli, L, et al. “Probing strong-field QED with Doppler-boosted petawatt-class lasers.” Physical review letters 127.11 (2021): 114801.
Abstract :
Relativistic electron beams have many potential societal applications, particularly in medicine. Today two types of accelerators are used to generate them: either linear accelerators, very bulky and expensive, or laser acceleration in a plasma, more compact and less expensive but not yet directly applicable for the general public. This has been a very active area of research for two decades with several challenges to overcome such as (i) increasing the charge (number of electrons), and (ii) improving the mono-energetic aspect of the beams.
Using a very high power femtosecond laser (a few 100TW) focused at Ultra-High Intensity (UHI), two types of acceleration were demonstrated:
- 1) by reflection of the laser on a solid target: thanks to the high density, a strong charge of several tens of nanocoulombs (nC) is accelerated but quite weakly, a few MeV, because on a small dimension (some 10-100 μm);
- 2) by propagation of the laser in a gas, a plasma bubble is formed in the wake of the pulse. It traps and accelerates electrons over several centimeters to reach very high energies, around 100MeV, but with a more modest charge of around ~1nC (low density gas). Gas cells with shocks were developed to inject electrons into the bubble in a very localized manner in order to make the beam quasi-monoenergetic.
At the Applied Optics Laboratory, we have demonstrated a new type of injection by combining a plasma mirror and a gas jet in order to combine the forces of the two systems, see figure, panel a.
- Step 1, injection: the laser reflects on a solid target in order to remove a large charge from the high density and this in a very localized manner, just on the surface of the target.
- Step 2, acceleration: then, the laser propagates a few millimeters in the gas to accelerate the electron bunch to high energies and monoenergetically. Experimental proof of such has been achieved very recently.
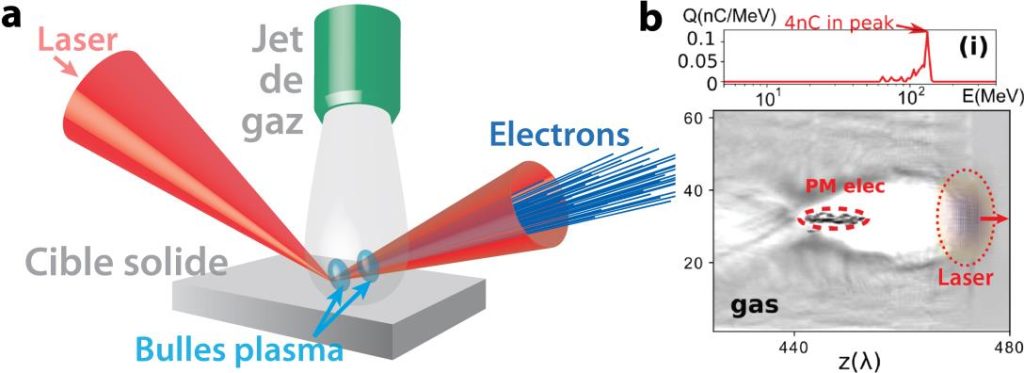
Objectives:
The electron beams generated by this new type of accelerator increase the performance of these laser-plasma accelerators tenfold and make it possible to consider medium-term applications. On the one hand, medical applications using lower energy but high cadence lasers, and on the other hand, the drastic increase in the electronic charge available on the largest laser installations in the world (PetaWatt laser) could allow to demonstrate for the first time experimentally the effects of Quantum ElectroDynamics (which predicts, for example, the creation of matter in a vacuum). These two axes will be studied during the thesis.
Internship/thesis objectives :
90% experimental, 10% theory/simulations. (i) The main objective is to adapt the experimental schemes developed at 100TW towards both 10TW lasers and PW lasers. Numerous experiments on the largest laser infrastructures in the world (APOLLON (Fr), ELI (Romania), BELLA (California)) will take place. (ii) Design the hybrid target with hydrodynamic simulations in order to minimize shocks during the expansion of the gas against the solid target. (iii) Carry out numerical simulations of the interaction in order to understand the different injection parameters and to determine which configuration makes it possible to optimize the acceleration conditions. (iv) Experiments on biological samples are planned on 10TW lasers.
Environment :
The experimental implementation and development parts of the hybrid target are carried out within the Ultra-fast Sources of Particles and X-rays (UPX) team at the LOA. Experiments on different lasers (Bordeaux, Marseille, California, Romania) will be carried out. A dynamic team that is at the world forefront in laser electron acceleration. The theory/numerical part is done in collaboration with CEA Saclay.
Possibility of thesis Yes
Probable contract-financing / Expected contract-funding? IPP Doctoral School
Contact :
State-of-the-art multi-PW lasers, such as APOLLON (France), and km-scale accelerators such as FACET-II at Stanford (USA) [1], allow to create on Earth the extreme conditions for fundamental interactions between particles and fields. Such interactions follow the laws of strong-field quantum electrodynamics (QED) that has emerged as a promising discovery science area with exciting opportunities. Strong-field QED effects appear when the electric field experienced by the electron in its rest frame approaches the Schwinger field ES=m2c3/(eℏ), and their most spectacular manifestations include the production of electron-positron pairs through the inverse-Compton scattering and the nonlinear Breit-Wheeler processes. At multi-PW laser facilities such as APOLLON, strong-field QED experiments can be performed by colliding high-energy electrons produced by a novel type of particle accelerator, namely a laser-driven plasma accelerator, with a high-intensity counterpropagating laser pulse or another source of strong fields. Several avenues will be explored in the ANR g4QED project (Gamma photon sources as a path for strong-field QED experiments), specifically including two promising concepts: (i) the use of a plasma mirror to reflect the laser pulse and enable the collision between the high-energy electrons and the laser pulse [2], and (ii) the use of multiple foils to focus the electron beam to very small size, and use the focused electron beam itself as the source of strong fields [3,4].
The internship and the PhD will aim to model, theoretically and numerically, these two concepts and to provide the best strategies to implement them experimentally on APOLLON and FACET-II. The work will involve a rich variety of physics: the interaction between laser pulses and plasmas, between particle beams and plasma, as well as the strong-field QED physics that we aim to unveil experimentally. The development of advanced numerical modeling for this project will also be key to provide accurate physical interpretation for the experiments. During the internship, the student will work more specifically on the first concept with the modeling of the plasma channel formation, the laser focusing and compression in the plasma, the laser-driven plasma acceleration, the generation of the plasma mirror for the laser reflection, and finally the strong-field QED collision. The goal is to understand its potential for strong-field QED experiments, as well as possible limitations or paths for further improvements.
[1] V. Yakimenko et al., Phys. Rev. Accel. Beams 22, 101301 (2019).
[2] K. Ta Phuoc et al., Nature Photonics 6, 308 (2012).
[3] A. Sampath et al., Phys. Rev. Lett. 126, 064801 (2021).
[4] A. Matheron et al., Comm. Phys. 6, 141 (2023).
PhD conditions: The M2 internship can be continued by a PhD with co-supervision by CEA and LOA, which will be funded by CEA through the ANR g4QED (collaborative project between CEA, LOA and LULI). In addition, the PhD student can apply to be Teaching Assistant at Ecole Polytechnique and other graduate schools.
Contact : Igor Andriyash / Sebastien Corde
PhD Thesis
When an intense laser pulse of femtosecond duration propagates in air or water, the appearance of numerous non-linear optical effects gives rise to filamentation, a spectacular process where part of the energy of the beam contracts to form a long channel in which the intensity is maintained at ~10^15 W/cm^2. These filaments make it possible to envisage applications such as the guidance of energetic laser beams or microwaves, the control of hydrodynamic flows in supersonic regime, the generation of UV laser radiation or THz pulses at a distance or finally the laser lightning rod .
One of the difficulties linked to the use of new very high power laser sources is that the filamentation process becomes highly unpredictable. Indeed, when the peak power of the beam exceeds a hundred Gigawatts, it gives rise to a multitude of filaments which develop through a modulational instability mechanism. Likewise, the increase in the rate of terawatt lasers gives rise to the appearance of thermal phenomena which strongly disrupt the propagation of the laser beam and the filamentation process.
This thesis work will initially consist of precisely characterizing these instability and thermal effects as well as their influence on the laser filamentation phenomenon. We will then seek to develop optical methods to control them and, if necessary, use them to improve the efficiency of the filaments in terms of secondary radiation sources (UV laser emission, THz plasma radiation, acoustic emission).
Link to apply
Laser-plasma electron acceleration offers a unique way to produce highly energetic and ultra-short electron bunches, on very short distances. It have risen much interest since the first, pioneering, experiments in the early 2000s (Malka 2002; Faure et al. 2004). The interaction between an intense laser pulse and a target material is responsible of the whole extraction, selection and acceleration process, which makes primordial the understanding of the role of the involved parameters, such as the target density, shape and profile, laser duration, phase and intensity.
Among the research fields which laser-driven particle sources are relevant for, radiation biology opens to the exploration of fundamental aspects of radiation toxicity on living matter, that will be accessible only with a radiation source as short as the physical dose deposition time (Bayart et al. 2019; Favaudon et al. 2000; 2014). In order to make laser-driven electron sources interesting and compatible with radiobiology applications, a number characteristics should be addressed, such as the total charge per accelerated bunch, the spectral features, the stability and the duration. The required improvements demand a deep understanding of the acceleration mechanisms, the design of novel acceleration strategies and schemes.
Throughout the thesis activity, high potential topics for fundamental and applied science will be addressed, in the field of laser-created plasmas, particle acceleration, particle detection or dosimetry and engineering of experimental systems towards applications.
keywords :Ultra-intense lasers, Laser-plasma interaction, Laser-driven electron acceleration, Numerical simulations, Ultra-high dose-rate
Contact : Alessandro Flacco / Cédric Thaury
Postdoc positions
Context
The appearance of high-power ultra-short laser systems at the end of the 1990s, and recent technological advances in diode-pumped solid amplifiers, now make it possible to envisage in the medium term the development of new applications of lasers. of femtosecond duration. Two key applications of these lasers are laser lightning control [1] and supersonic flow control [2] which are based on the phenomenon of femtosecond laser filamentation in air [3].
This project consists of studying the use of femtosecond laser filaments to produce a “virtual” plasma antenna emitting in the RF range [4]. To do this, it will be necessary to enrich the plasma column initially created by the femtosecond laser pulse using a high-voltage generator [5] or a power microwave source [6]. The two methods will be tested experimentally in the LOA premises and the plasma antenna will be characterized using various diagnostics (rapid imaging, interferometry, spectroscopy, etc.).
Summary of work
The candidate will be required to characterize the plasma column generated by laser filamentation using plasma spectroscopy and interferometry methods. He/she will then seek to couple energy into this filament by means of high-voltage discharges, nanosecond laser pulses and power microwaves in order to increase the conductivity and lifespan of the column. plasma. Finally, he or she will participate in an experiment guiding electric discharges by laser on a megavolt power Marx generator. This work will be carried out in collaboration with ONERA Palaiseau as part of a DGA study.
The candidate must have knowledge of plasma physics, optical or plasma diagnostics, and notions of optics.
Net monthly salary: between 2500 and 3000 euros depending on experience.
Contract duration: 2 to 3 years.
Planned start: February 2025
The work being carried out within the framework of a DGA contract, the candidate must have French nationality.
Contact: aurelien.houard@ensta.fr
Team posts related to the topic
1. Laser-guided lightning, Nature Photonics 17, 231 (2023)
2. Improving supersonic flights with femtosecond laser filamentation, Science Advances 4, eaau5239 (2018)
3. Femtosecond filamentation in transparent media, Phys. Rep. 441, 47 (2007)
4. Radiofrequency plasma antenna generated by femtosecond laser filaments in air, Appl. Phys. Lett. 101, 264106 (2012)
5. Study of consecutive long-lived meter-scale laser-guided sparks in air, Physics of Plasmas 30, 083503 (2023)
6. Transfer of microwave energy along a filament plasma column in air, Appl. Phys. B 123, 40 (2017)
Context :
Laboratoire d’Optique Appliquée (LOA) is developing a new 3D X-ray imaging technique, called plenoptic, based on the combination of main optic and wavefront sensor. Three systems have been built. The first one was a demonstrator running at PETRA III synchrotron in Germany at an energy of 11 keV. It is now disassembled after producing excellent results. Another camera is set and aims at imaging living biological cells. It is working around 400 eV. The last system is targeting small animal imaging with X-rays of energy around 17 keV. Both systems are tabletop.
Both systems still need to be fully tested and improved. The low energy camera is running at LOA, near Paris, while the high energy system is set at Imagine Optic company in Bordeaux, France. LOA’s team is in charge of running and improving both systems through a collaboration agreement.
Today, we are using three software: those specifically developed for the so-called focused ad unfocused plenoptic cameras and a homemade software integrating the two geometries. These softwares are too slow and complex for generating a 3D image.
Topic of the post-doctoral fellowship:
A post-doctoral position is open in FLUX group for 18 months with possibility of extension. During this period, the post-doc will be in charge of analyzing ability of machine vision software to generate efficiently a 3D image from the raw plenoptic data. Disparity as well as machine learning are two options we consider. The candidate will have to choose one or different techniques, implement it/them and then test it/them on real X-ray plenoptic images.
Experience :
Candidate should have developed strong computational skills related to machine vision. Good knowledge on PYTHON is a plus but not required. The candidate should be rigorous and have a proficiency in working in team. English is the work language.
Contact : philippe.zeitoun@ensta.fr
Context :
Laboratoire d’Optique Appliquée (LOA) is developing a new 3D X-ray imaging technique, called plenoptic, based on the combination of main optic and wavefront sensor. Three systems have been built. The first one was a demonstrator running at PETRA III synchrotron in Germany at an energy of 11 keV. It is now disassembled after producing excellent results. Another camera is set and aims at imaging living biological cells. It is working around 400 eV. The last system is targeting small animal imaging with X-rays of energy around 17 keV. Both systems are tabletop.
Both systems still need to be fully tested and improved. The low energy camera is running at LOA, near Paris, while the high energy system is set at Imagine Optic company in Bordeaux, France. LOA’s team is in charge of running and improving both systems through a collaboration agreement.
Topic of the post-doctoral fellowship :
A post-doctoral position is open in FLUX group for 18 months with possibility of extension. During this period, the post-doc will be in charge of finishing to set the two systems, test them and then optimizing them. On both cases, the main objective consists in generating 3D images of adequate samples in a single exposure. Known samples like USAF 1951 will be used first; we will move later to biological samples provided by our collaborators. The measurement of the dose delivered to generate a 3D image will be performed at every step. Most interesting samples will be imaged by X-ray computed tomography and results will be compared to those obtained by X-ray plenoptic.
Experience :
Candidate should have a strong background on experiment in optics or using complex optical systems. Knowledge on X-rays, Optical design software (ZEEMAX, OSLO etc) or PYTHON is a plus but not required. The candidate should be rigorous and have a proficiency in working in team. English is the work language.
Contact : philippe.zeitoun@ensta.fr